Learning How to Make Mini-Proteins that Bind to Specific Target Proteins
Advisor: David Baker (Biochemistry)
Antibodies seem to have no trouble targeting and binding to specific natural proteins. But how do they do it? Human attempts to copy this ability often directly copy antibodies; either by borrowing their loops or their hotspots. While humans can analyze interactions between antibodies and their targets, or interactions between native protein complexes, the proof of understanding requires one to make and test predictions. In this work, we set out to learn the principles that allow for specific protein binding. Leveraging the modern biochemist’s toolkit, we tested more than one million de novo mini-protein binders (< 65aa) to more than twenty natural targets. With the goal of learning, not copying, we threw out the native complex information and discovered our own hotspots and interactions. Some of the target sites did not even have natural partners. By iterating on design-test-learn, we were able to uncover secrets about protein folding and protein binding. We found that the hydrophobic effect and hydrophobic packing dominate the correlations. While future work may find ways to bind to polar patches, for now, we have a sure-fire way to make binders to hydrophobic patches.
Brian will continue on in the Baker lab as a research scientist at the Institute for Protein Design.
Zwitterlation improves efficacy and safety of therapeutic protein
Advisor: Shaoyi Jiang (Chemical Engineering)
During the past decades, engineered proteins have been widely used as biopharmaceutical drugs (e.g., recombinant therapeutic proteins, enzymes, and monoclonal antibodies) and gene editing tools (e.g., zinc fingers, TALENs and CRISPR/Cas9). This has significantly transformed the pharmaceutical industry. Compared with conventional small molecule drugs, these biomacromolecules offer the advantages of higher specificity and potency. However, safety and efficacy concerns have been raised from different aspects. In her thesis, Yanjiao discusses the tactics to improve efficacy and safety of protein therapeutics. First, to protect the protein from body clearance and immune system, a poly(carboxybetaine) (pCB) polymer is conjugated to the protein surface. Compared with PEG conjugation, the zwitterionic coating more effectively shields protein epitopes, rendering the whole protein invisible to the body immune system. This strategy is proven to be effective in increasing protein stability, prolonging in vivo circulation half-life and reducing immunogenicity, and is successfully applied to interferon-alpha (IFN-alpha). Compared with PEGylated IFN-alpha, Zwitterlated IFN-alpha largely retains its in vitro efficacy. In addition, the author further discusses the tactics to improve the safety and efficacy of nuclease for gene editing. To reduce the off-target effect of CRISPR/Cas9 system, Yanjiao discussed a novel method that conjugating CRISPR/Cas9 with zwitterionic pCB polymer to address the mismatch issue at the off-target sites. Yanjiao demonstrated the conjugation of CRISPR/Cas9 system with pCB polymer can both increase the efficacy of Cas9 nuclease and reduce non-specific interactions between gRNA and DNA (off-target DNA break). Finally, we converted the strategy to the mRNA version via a new strategy for reducing off-target effect of CRISPR/Cas9 systems that relies on fusing zwitterionic peptide poly(EK) extensions to the terminus of Cas9 via genetic engineering. The poly(EK) peptide is biodegradable and potentially safer for clinical applications. This technology was realized through mRNA delivery and will provide a robust and easily used strategy to reduce "off-target" mutations on a genome-wide scale and to improve the efficiency of a wide range of CRISPR/Cas9-based biological and clinical applications.
Yanjiao is now pursuing a career in industry.

Molecular Engineering of Peptide-Inorganic Interfaces: From Aqueous Conformations to Complex Surface Assemblies
Advisors: Rene Overney (Chemical Engineering) / Mehmet Sarikaya (Materials Science & Engineering)
Bio-inorganic interfaces, in which biomolecules intimately contact inorganic materials, have become the centerpiece for advanced technologies in medicine, catalysis, and electronics. Considering the convolutional complexity of interfaces involving environmental conditions and biomolecular and inorganic surface intricacies, it is of utmost importance to obtain a fundamental understanding of molecular conformations, interactions, and hybrid interfacial properties. Based on such basic understanding, it becomes feasible to engineer molecules with the tailored ability to spontaneously build-up, via self-assembly, a bio-inorganic interface with desired device relevant properties. It has been recognized in this Thesis that solid-binding peptides are suited perfectly for this molecular engineering task due to their vast sequence space, labile conformational nature, and reliance on soft intermolecular interactions that can be fine-tuned through environmental controls, and ability to form long-range ordered structures. Here, solid-binding peptides designed to bind and assemble on graphite surfaces are interrogated using scanning probe microscopy techniques and molecular dynamics simulations to explore and control their self-assembly pathway. Thermal selection of peptide conformations is shown to direct the long-range ordering of peptides at graphite surfaces. Through energetic analysis of peptide-graphite interactions, using a technique dubbed Intrinsic Friction Analysis, the molecular implications of thermal conformational selection are elucidated and used to rationally design a peptide with tailored binding energy and assembly structure. The impacts of these thermally selected conformations on electron transport across the bio-inorganic interface are interrogated via scanning tunneling spectroscopy and metadynamics, revealing control over device relevant properties. Peptide-substrate recognition is explored using atomic resolution microscopy to understand peptide miscibility and nucleation in binary assemblies. Finally, the role of lateral confinement on self-assembly is explored, revealing unexpected peptide adsorption and assembly phenomena enabling tailored self-assembly at various length scales. From these fundamental insights, novel bio-inorganic devices can be rationally designed for targeted technological applications.
Tyler is now a postdoc in Professor Stuart Rowan’s lab at the University of Chicago’s School of Molecular Engineering working on developing peptide/polymer materials with mechanoresponsive properties.
Nanopore-based SARS-CoV-2 helicase inhibition assay and De novo design approaches for nanopores with diverse architectures
Advisors: David Baker (Biochemistry) / Jens Gundlach (Physics)
Nanopore technologies harness the atomic precision of transmembrane proteins to create a highly confined channel within which to study molecules at the angstrom-scale in real-time. In my research, I explored de novo design principles to procedurally generate diverse channel geometries with Rosetta and studied their ability to form pores within membranes. Our ability to create customized pores is a step towards enhancing the accuracy of nanopore DNA sequencing and single-molecule identification.
While a precise nanopore allows greater discrimination between DNA bases, yet another protein is responsible for the controlled release of DNA into the pore. Nanopore DNA sequencing relies on enzymes that bind and walk on DNA to slow the rate of DNA translocation from the speed of ions to that of the enzymes themselves. This in turn gives access to the kinetics of the enzyme at the microsecond timescale. In my research, I also show our ability to monitor the SARS CoV-2 helicase walking in single-nucleotide steps and its inhibition. This forms a basis to study how viral inhibitors target the helicase and provide insights for their development.
Sinduja will continue in the Gundlach lab as a postdoc while wrapping up several projects.
Computational Discovery of Novel Secondary Structures from Non-Canonical Amino Acids
Advisor: David Baker (Biochemistry)
Macromolecules, and more specifically proteins, are ubiquitous among biological processes, and these macromolecules are composed of fundamental components known as primary and secondary structures. Proteins have naturally evolved to adopt an impressive myriad of functions that are critical for life, and humanity has recognized that the development of new proteins with new functions that solve modern challenges is an important goal. However, while progress has been made toward this goal, the rational engineering of proteins remains a grand challenge because proteins evolved without the intention of being rationally engineered. Therefore, the development of new macromolecules which outperform proteins in key metrics like programmability, stability, and diversity could accelerate the efforts to engineer and, more importantly, utilize designed macromolecules. In my thesis, I describe my development toward designing these new macromolecules. This work focused on the identification of new fundamental components of macromolecules, primary and secondary structure, to find new and better building blocks for future molecular engineering efforts. The identification of candidate molecules, which are composed of non-canonical amino acids, was performed computationally. New methodologies were developed to effectively search this previously unexplored, theoretical space. Finally, once promising molecules were identified from the computational studies, the molecules were experimentally characterized to determine the accuracy of the predictions.
Adam is currently wrapping up several research projects in the Baker lab.
Understanding Cell State Transitions in Development and Disease
Advisor: Hao Yuan Kueh (Bioengineering)
During development, eukaryotic cells undergo a series of state transitions that transform a stem cell into a diversity of differentiated progenitors with distinctive biological functions. Disruption of such processes can drive cancer and autoimmune diseases. Yet it is largely unknown what molecular mechanisms can lead to maintenance and transition of cellular states and how scientists can effectively identify and monitor these states in novel biological systems. This thesis aims to address these challenges using hematopoietic cells as a model system. I started by investigating the epigenetic mechanism controlling activation timing of the fate determining gene Bcl11b by a histone-based mechanism during early T-cell development. I then explored the potential of such epigenetic mechanisms to generate diverse schedules and patterns of population size control during development. Finally, I designed a machine learning workflow method for the discovery and exploration of phenotypic states and their dynamics from brightfield movies of unmanipulated cells. This technique is then applied to identify stem-like and differentiated phenotypic states in primary patient acute myeloid leukemia stem cells during differentiation.
Sam is currently pursuing a postdoc in southern California.
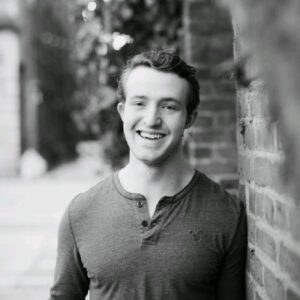
Determination of alpha-sheet secondary structure as an early biomarker in the pathogenesis of Alzheimer's Disease
Advisor: Valerie Daggett (Bioengineering)
Alzheimer's disease (AD) is one of over 50 amyloid diseases discovered to-date and is now the sixth leading cause of death in the United States, affecting over 5.8 million Americans and projected to cost upwards of $1.1 trillion in paid and unpaid care by 2050. It is characterized by the deposition of beta-sheet-rich, insoluble amyloid beta-peptide (Abeta) plaques; however, the presence of toxic soluble oligomers --not plaque burden -- is correlated with cognitive impairment in AD patients. Additionally, plaque deposition is a late-stage indicator of disease, while the early-stage oligomers mediate toxicity 10-20 years before symptoms present. Most existing treatments for AD target and alleviate symptoms of the disease, rather than the early stages of pathogenesis. Additionally, current diagnostics either detect late-stage indicators of disease progression or utilize biomarker levels that are not directly correlated with toxicity in AD pathogenesis. In this thesis, we show that Abeta oligomers adopt a nonstandard secondary structure, termed alpha-sheet, in the early stages of aggregation that is strongly correlated with toxicity. De novo designed alpha-sheet peptides specifically and tightly bind the toxic oligomers over monomeric and fibrillar forms of Abeta. Alpha-sheet peptides inhibit aggregation in vitro, neurotoxicity in cell culture, and remove toxic oligomers from the brains in vivo in transgenic (Tg) mice. We further developed and characterized a novel diagnostic agent, termed the soluble oligomer binding assay (SOBA), that specifically detects alpha-sheet containing Abeta oligomers in solution. SOBA detected toxic Abeta oligomers in the brains of Tg mice long before plaques deposited or symptoms presented, and correctly identified all mild cognitive impairment (MCI) and AD patients in a cohort using plasma samples. SOBA also correctly identified all control (CO) patients (including some with non-AD-related MCI) with an overall sensitivity of 100% and specificity of 100%. Additionally, 8 pre-symptomatic CO patients that converted to MCI within 1-5 years were correctly identified by SOBA before clinical assessments or biomarker characterizations could. The alpha-sheet hypothesis proposes the earliest mechanism through which AD pathology initiates and propagates, presenting the possibility for novel therapeutic agents for efficacious treatment of AD.
Dylan is now a principal scientist at AltPep, a Daggett lab spinout that is developing early diagnostics, modifying therapeutics and anti-biofilm treatments for amyloid diseases.
Computational Design of Tunable Kinetic RNA Biosensors
Advisor: James Carothers (Chemical Engineering)
Bacterial metabolism is made up of complex gene networks that can be engineered to produce valuable chemicals from renewable resources such as sugar. However, these complex networks require sophisticated genetic engineering to optimize the production of these high-value compounds. For most biosynthetic pathways, it remains impossible to predict what combinations of gene expression levels will give rise to the most productive bacteria. Therefore, to identify the most productive pathway variants it is necessary to develop biosensors that can give a visible readout of how much of the target chemical each cell is producing. In nature, bacteria’s solution to measuring the concentrations of important chemicals is a class of RNA based biosensors called “riboswitches”. Riboswitches bind their target chemical and modulate gene expression levels in response. However, despite numerous design and selection methods published to date, there exists no common methodology to engineer high-performance, tunable, chemical-responsive RNA biosensors from first principles. By combining design lessons learned from nature with novel kinetic RNA folding predictions we present a new class of engineered RNA biosensors with increased activation ratios and tunable ligand sensitivities, both of which are essential for applying biosensors to real-world problems.
David recently co-founded a startup company with MolE graduate and fellow Carothers lab alum Jason Fontana.
Quantum dot probes for neuroimaging and visualization of extracellular vesicles
Advisor: Elizabeth Nance (Chemical Engineering)
Central nervous system (CNS) diseases have long known hard to treat due to (1) highly restricted barriers such as the blood brain barrier (BBB) and the brain parenchyma penetration barrier, and (2) the dynamic and heterogeneous neurological disease microenvironment, which is dependent on disease etiology and disease progression, and is variable from patient to patient. Nanotechnology based imaging probes, such as quantum dots (QDs), are thus of interest to study complex changes in the brain and develop cutting-edge imaging tools. QDs have several advantages over traditional probes including tailorable excitation and emission spectra, tailorable surface functionalities, and high photoluminescence and photostability, which are ideal characteristics for in vivo imaging. QDs have been utilized in various applications in the brain; however, a systematic evaluation of QD behavior in brain-relevant conditions has not been done. Therefore, we first sought to comprehensively investigate QD colloidal stability, toxicity, and cellular uptake in vitro, ex vivo and in vivo in the neonatal brain environment. We found QD behavior is highly dependent on surface functionality, and QDs can activate metallothionein detoxification pathways in cells in organotypic whole hemisphere slice cultures. QDs are mainly internalized in the corpus callosum region in microglia, cells that mediate inflammation in the brain. Using multiple biological models in this study also indicated the need for careful consideration of barriers that exist in different study platforms, when investigating nanomedicine behavior in brain microenvironment. We next sought to utilize QDs to label and visualize extracellular vesicles (EVs). EVs are cell-secreted vesicles that play an important cell-cell communication role and participate in normal and pathological processes. Direct visualization and tracking of EVs is essential to broaden the utility of EVs as biomarkers of injury, however, current labeling techniques fail due to lack of sensitive, specific and robust labeling. We have developed a QD-EV conjugation platform using 4-formylbenzoate (4FB) to 6-hydrazinonicotinate acetone hydrazone (HyNic) click chemistry. We showed this method can be performed under physiological conditions, is universal to EVs coming from different sources, such as human semen fluids and rat brain, and has better resistance to photobleaching compared to commonly used labeling dye. This QD-EV conjugation method also has room for optimization by using catalyst or altering QD:EV ratio. QD-EV conjugates and unconjugated QDs can be easily separated by size exclusion chromatography (SEC). We also show this QD-EV conjugation platform enables high resolution visualization and real-time study of the EV-cell interaction. In summary, we investigated both fundamental understanding of QD neuroimaging probes in the developing brain and application of QDs for labeling and visualizing EVs. The understanding of QD behavior and impact in the brain, and subsequent use of QDs to label and trace biological entities represents a promising advancement in the field of bioimaging for neurological disease.
Mengying is now a postdoc in the lab of Dr. Viviana Gradinaru at Caltech developing new tools to understand and repair the nervous systems via targeted gene therapy.